The collision of high energy particles with the sky in the polar regions to create the stunning aurorae borealis and australasis are easy to visualise and bring to mind. The hot pixels in some CCDs and other ionisation events tell us that high energy cosmic rays fly through the atmosphere, reach the ground and do some level of damage. This is another high energy particle shower similar in effect to the aurora, though involving higher energy particles from different sources (directly from the Sun and the Galaxy). Even the fact that solar radiation ionises the atmosphere and the electrons liberated are sometimes capable of exciting and ionising further gases can be imagined. But there are further occasions when sub-auroral signals can be created through acceleration of the particles in the magnetosphere or even just the way the magnetic field is orientated.
Offset and into the atmosphere
To a good approximation, the field near to the Earth is a dipole, similar to the magnetic field of a bar magnet. However, the axis of the field isn’t exactly where the rotational axis of the Earth is, it is offset by about 11.3 degrees from that line. As well as being tilted to the axis of rotation of the Earth, the centre of the magnetic field isn’t where the centre of the Earth is, it too is a little offset – by about 450km. As a result, the area above the South Atlantic is higher up from the centre of the magnetic field than any other place on Earth, which means the atmosphere here is further out into the magnetosphere. This is the South Atlantic Anomaly.

Earth's magnetic field
Within the region over which the Earth’s magnetic field is dominant, many particles are trapped. They are forced to travel along the magnetic field lines by electromagnetic forces acting on them. They slow down as they move towards the Earth, eventually stopping and turning back at a ‘mirror point’. Unless something happens to disturb them, they will remain bouncing from point to point on the field lines. The Van Allen Radiation Belts and the Plasmasphere are two areas where a large concentration of charged particles remain.
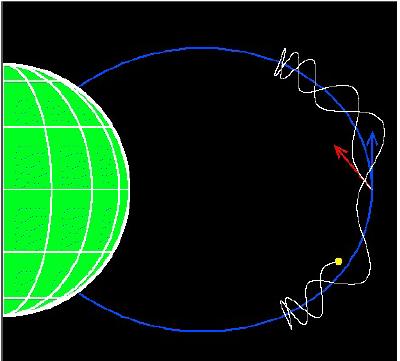
Bounce motion of particles on field lines
However, the particles will move a little. Further electromagnetic forces acting on the particles will cause them to move east or west from field line to the next field line in what is called drift motion. The slow orbit around the Earth brings some of them to the South Atlantic Anomaly, where the higher than usual atmosphere allows collisions to happen between it and the trapped particles. Below is an image of particle concentrations across the Earth at a certain altitude. You may wonder then why there aren’t permanent aurorae above the South Atlantic. Well, there are in a way, but they are just very faint. The particles rather than being injected directly into the atmosphere to dump all their energy, as they do in the poles, just scrape the atmosphere and return to the magnetosphere, giving one or two collisions rather than hundreds or thousands. Although there’s lots of particles doing this, they end up transferring so little energy that it doesn’t provide much of a signal.

Particle populations at 560km by ROSAT
Delivering a punch
I spoke above about trapped populations of particles – now how do they get trapped? I mentioned the bounce effect above, but for this to happen, the particles have to leave the atmosphere and actually get trapped in the field line. The reason for this is whilst a proton or an electron is in the atmosphere, then the forces created by bumping into other particles will be stronger than the electromagnetic forces that want the charged particle to follow the field lines. For the following to happen, something has to get the charged particle to an altitude where the atmosphere has such a small density that the collisions which can send them in other directions just stop happening. Below is an image of particle input and output in the polar regions of the Earth.

Polar particles; precipitated and passed-out
Things going in include solar radiation (light, x-rays, radiowaves), auroral particles, fast solar wind particles (entering via the cusp), slow solar wind particles (the polar rain). In addition to these, cosmic rays also come in to say hello. These create heating and ionisation. There is also a current system accelerating things in and a few things out (Birkeland currents) during auroral events. Thermal velocities depend on mass, electrons are tiny little things and though protons are far larger, they’re still smaller than the average atmospheric particle. This means they get more heat energy from the other parts of the atmosphere and they move faster when they have it. This in turn allows electrons to pile out of the atmosphere at the escape velocity and into the magnetosphere.
A lot of the electrons will therefore quickly become trapped on the field lines, but as the magnetic (and even the rotational) axis isn’t aligned with the day-night terminator, some dayside electrons shot straight up the field line will come straight down in the nightside in a place where things expect to be cooling down and recombining after the sunlight switches off. This too enhances the airglow to a subauroral amount. Not enough to cause visible emissions, but enough to warm the atmosphere in otherwise unexpected places.
Radio gaga
Another thing you’ll see on the diagram above are points where ULF/VLF radiation is emitted by the Earth (Ultra or Very Low Frequency – high wavelength waves). Photons are electromagnetic waves and if one finds itself with either the electric or magnetic vector aligned with the Earth’s magnetic field, it can become trapped and ride the field line to the other end. These are known as P and Q waves and are important as they can accelerate or scatter trapped electrons – this can then cause trapped electrons to come down and strike the atmosphere. These waves accelerate by allowing the electrons to effectively surf on them as they move.
It is believed that this effect causes quite a strong additional source of ionisation in Jupiter’s atmosphere as well as one in the Earth’s (it has been measured in the South Atlantic Anomaly, the best place to see it happening as the scattered or accelerated electrons don’t require as much scattering or accelerating to get there).
Although these are all very minor effects, ionisation and heating affect wind and chemistry and a good atmospheric model of any planet will keep an eye on their inputs – even to this level.